“Energy to Get the Job Done and Lipoic Acid Mineral Complex”
DNA-Energy.jpg
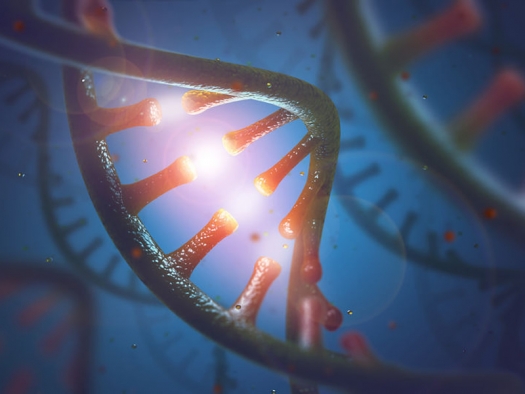
Image by ktsdesign / 123rf.com
Over fifty years ago, most scientific and medical therapeutic approaches focused on cellular metabolism. With the advent of genetics a concentrated shift toward genomics, and subsequently proteomics (protein profiles), dominated the therapeutic stage. The area of metabolism (metabolomics) is now being revisited as an attractive target.
One such regulatory approach is via the manipulation of cellular energy. Cellular energy is synonymous with metabolic power. As we age there is a decrease in metabolism, furthermore, numerous disease states involve metabolic dysfunction (i.e. ischemia/stroke, cancer). As we all know, the major power plant of the cell is the mitochondria. It utilizes high energy intermediates (NADH and FADH) to donate electrons and drive the production of ATP, our functional energy source. Can we alter metabolic fitness by providing an alternative electron source?
What is Poly-MVA?
Poly-MVA is a dietary supplement that contains a patented lipoic acid mineral complex (LAMC). LAMC is composed of the antioxidant lipoic acid, the mineral palladium irreversibly bound in a trimer about thiamine (B1). The name Poly-MVA was coined by Dr. Albert Sanchez since Poly MVA is composed of minerals, vitamins, and amino acids (MVA). In addition to the LAMC complex, the proprietary formulation contains riboflavin, cyanocobalamin, formyl-methionine and acetyl-cysteine.
The LAMC family of compounds was discovered by Dr. Merrill Garnett. Dr. Garnett was formally trained as a dentist; this was followed by substantial graduate work in biochemistry and electrochemistry, followed by over 40 years of scientific bench work. His inquiry and screening into thousands of organo-mineral compounds, led to the discovery of the safe and effective complex and chemotherapeutic agent via its metabolic concept of redox signaling. The principles that led to this finding in the early 90s still drive Dr. Garnett's principle laboratory interests today; that ultra-low frequency electrical currents are at the heart of all physiological processes and determine such events as the polarization, charge and folding of enzymes, nucleic acids and membrane phospholipids. He has shown that the regulation of charge transfer can form the basis of several new methods of medicinal cellular management.
Mechanisms of action
Poly MVA’s researched mechanisms of action is directly related to its structural formulation of the Lipoic Acid Mineral Complex. Dr. Garnett’s complex is a liquid crystal polymer and provides a unified redox reaction. Redox polymers more efficiently accept charge, and therefore serve as potent anti-oxidants while also having the ability to donate this charge. In the case of LAMC it serves as alternative energy sources at an extremely high level increasing mitochondrial efficiency, repair so that enhanced cellular functions can take place. This high energy electron transfer has been studied and tested to be the key to its physiological effectiveness.
When glucose enters a cell it is broken down under anaerobic conditions (absence of oxygen) into pyruvate. Pyruvate subsequently enters the mitochondria, via complex I, and is quickly oxidized, in the presence of alpha-lipoic acid, to acetyl-CoA. In aerobic (with oxygen) respiration, acetyl-CoA is then channeled into the Krebs/Citric Acid Cycle to create the reduced form of nicotinamide adenine dinucleotide (NADH). NADH donates its electron to the electron transport chain to drive the phosphorylation of adenosine triphosphate (ATP). The energy needs of the body are supplied by splitting ATP into adenosine diphosphate (ADP) and a free phosphate molecule.
Studies have demonstrated that LAMC provides electrons to DNA (to replace the electrons lost in normal cells as a result of the oxidative damage associated with radiation and chemotherapy) via the mitochondria. This electron transfer will provide an additional energy source to normal cells. However, cancer cells are metabolically challenged and function in a hypoxic (low oxygen) environment. Since excess electrons have less oxygen to accept them in the cancer cell, a local generation of free radicals occurs at the mitochondrial membrane. This activates apoptosis (cell death) by facilitating the release of cytochrome C from the inner mitochondrial membrane, allowing the formation of an apoptotic complex in the cytoplasm. This complex results in the subsequent activation of the caspase cascade of enzymes that destroy the malignant cells. Given that healthy cells are richly oxygenated, LAMC can increase the energy cascade and these cells can actually benefit from the energy boost.
Research findings have focused on the role of LAMC and an abnormal cell’s ability to physiologically adapt to a hypoxic environment. These physiological changes are mediated by a molecule called HIF-1 (hypoxia inducible factor-1), which increases in hypoxic conditions to promote increases in: Vascular Endothelial Growth Factor (VEGF) - a promoter of angiogenesis; Glucose Transport 1 (GLUT1) and glycolytic enzymes – critical components in anaerobic respiration; and Erythropoietin (EPO) – responsible for the differentiation of red blood cells. LAMC has been measured to decrease the production of HIF-1 thus restricting the ability of the cells to adapt to its environment and subsequently making it more vulnerable to the apoptotic death discussed above and is showing to be complimentary in various protocols and approaches.
Lipoic acid mineral complex modulates free radicals in neurodegenerative diseases
Oxidative stress is caused by the chemical imbalance between reactive oxygen species (ROS) production and their breakdown by reducing agents or antioxidants. Over-abundance of ROS has been found during neuronal development, as well as in numerous neuropathological conditions. A predominant feature of neuronal injury is the onset of oxidative stress [Higgins et. al., 2010].
Oxidative stress and mitochondrial dysfunction have been closely associated in many sub-cellular, cellular, animal, and human studies of both acute brain injury such as ischemia and stroke and neurodegenerative processes such as Parkinson’s, Alzheimer’s and Huntington’s. While the oxidative stress occurs chronically in Alzheimer’s disease, it is more acute in ischemic reperfusion injury. The consequences of mitochondrial dysfunction include DNA and protein damage, lipid peroxidation, disruption of the mitochondrial permeability transition, Ca2+ homeostasis, and triggering apoptosis. It is essential to have a healthy mitochondria contributing substantially to the physical, mental, and emotional elements needed to support the well-being of patients suffering from brain injury or neurodegenerative diseases [Krishnan et.al., 2011, 2012].
Energy metabolism, calcium regulation, and apoptosis-signaling pathways are the major roles of mitochondria. Energy requirements dictate the number of mitochondria in a cell [Alberts et. al., 1989; Voet D. & Voet J. G., 1995; Beattie, 2002; Nagley et. al., 2010]. Cardiac and skeletal muscles, the brain, and the liver have the most mitochondria because of their high metabolic activities. These cells are also exposed to the most oxidative stress because the source of free radical production is also the mitochondria. Due to low levels of antioxidants in neurons, they are intrinsically ill-equipped to defend against an increase in oxidative stress. Glial cells including astrocytes play a supplementary role in antioxidant defense of neurons [Higgins et. al., 2010].
Our search for an extremely safe (up to 40 mL/day, 0.037 M aqueous solution) and effective therapeutic agent resulted in the development of this novel redox molecule, “Lipoic acid mineral complex” that is active in mitochondrial cellular metabolism and other functions. The selection of the naturally occurring coenzyme, α-lipoic acid, as our ligand was based on its safety as well as its redox, antioxidant, and fatty acid properties. After selecting the ligand that plays a critical role in biological energy metabolism and numerous other functions, we wanted to tweak the properties of the ligand by complexing it with a mineral that is safe and has very high catalytic and electronic properties. After numerous investigations with a variety of minerals, the final selection was made to use palladium, a transition mineral.
The properties of the resulting lipoic acid mineral complex were remarkable in many ways and have been reviewed recently [Krishnan et. al., 2011]. Briefly, this complex enhances the enzymatic activities of Krebs cycle enzymes, isocitrate dehydrogenase, α-ketoglutarate dehydrogenase, succinate dehydrogenase, and malate dehydrogenase and mitochondrial respiratory enzymes, complex I, complex II, complex III, and complex IV. These enzymatic activity enhancements by the metal complex were, in general, much greater than that of the ligand, α-lipoic acid. Coupling this increase in the efficiency of the aerobic metabolic cascade with its powerful antioxidant properties, such as scavenging of free radicals, lowering lipid peroxidation, increasing the levels of glutathione, glutathione peroxidase, manganese superoxide dismutase, and catalase, gave us a powerful weapon to combat fatigue associated with numerous mitochondrial abnormalities [Sudheesh et al., 2009, 2010; Ajith , 2014]. The complex also modulates mitochondrial dysfunction, acts as a prophylactic for neuronal protection from transient ischemic attack, repairs DNA damage resulting from radiation, acts as a prophylactic for protection from radiation, and improves the quality of life. The therapeutic ability/potential of this complex may be exploited in its applications for combating brain injury resulting from transient ischemic attack, death of neurons and other progressive loss of structure or function of neurons associated with diseases such as Parkinson’s, Alzheimer’s and Huntington’s.
Mitochondrial dysfunction in neurodegenerative diseases
Since different organs can rely on mitochondrial energy to different extents, mitochondrial defects can cause organ-specific phenotypes. The organ system most reliant on mitochondrial energy is the central nervous system. The consequences of mitochondrial dysfunction are numerous and include oxidative stress, loss of cellular Ca2+ homeostasis, promotion of apoptosis, and metabolic failure. Hence, evidence continues to accrue implicating mitochondrial dysfunction in the etiology of a number of neurodegenerative conditions such as Parkinson’s, Alzheimer’s, and transient ischemia.
In transient ischemia, a lack of oxygen and glucose delivery compromise the integrity of aerobic metabolism, while reperfusion potentiates injury via the generation of free radicals. Superoxide, nitric oxide and peroxynitrite production in the brain is increased during reperfusion following 30 minutes of global ischemia. In patients with Parkinson’s disease, excess Fe2+ can reduce peroxide and produce hydroxyl radicals. These radicals and their reactions cause oxidative stress and consequent mitochondrial damage resulting in mutations. Evidence for mitochondrial dysfunction in Alzheimer’s disease pathogenesis comes from impaired activities of three key Krebs Cycle enzyme complexes and reduced respiratory chain complex I, III, and IV activity observed in postmortem Alzheimer’s disease brain, and oxidative damage to both mtDNA and nDNA.
Apart from ATP production, mitochondria also produce reactive oxygen species (ROS). Some electrons escaping or leaking from the electron transport complexes, mainly from complexes I and III, during respiration react with oxygen to form superoxide radicals. However, it may be possible for mitochondrial DNA (mtDNA) mutations to disrupt the normal electron flow and seriously affect energy production. Oxidative damage and the resulting serious consequences have been extensively reviewed recently [Singh, 2006]. Compared to nuclear DNA (nDNA), mtDNA is far more susceptible to mutations due to their being present in a highly oxidative environment, a lack of protective histones and limited repair capacity [Carew & Huang, 2002; Singh, 2006]. In aging and neurodegenerative disorders, apart from inherited defects, mDNA deletions and point mutations within neurons are well recognized.
At the heart of a number of neurodegenerative disorders is mitochondrial dysfunction. Lipoic acid mineral complex is formulated to combat mitochondrial dysfunction. The unique electronic properties of LAMC can now support proper mitochondrial function.
Lipoic acid mineral complex facilitates aerobic metabolism much more than that of α-lipoic acid, by significantly enhancing the enzymatic activity of isocitrate dehydrogenase, α-ketoglutarate dehydrogenase, succinate dehydrogenase, and malate dehydrogenase at the Krebs cycle and mitochondrial complexes I, II, III, and IV of the electron transport chain. The lipoic acid mineral complex formulation enhances the activities of catalase and glutathione peroxidase more than that of α-lipoic acid. The level of glutathione also was significantly improved, and the level of lipid peroxidation was decreased in the heart mitochondria of aged rats.
Lipoic acid mineral complex formulation is similar to a multi-spectrum drug. Since it targets the mitochondria it is able to carry out several functions such as combating age-related as well as disease-associated fatigue and minimizes the effects of ischemic injury. Being a powerful free radical scavenger, it may also be effective in combating death of neurons and other progressive loss of structure or function of neurons caused by free radicals.
Hypoxia: Low or lack of oxygen
Hypoxia is a state induced by the long or short term reduction in oxygen delivery to vital tissues. Low oxygen environments are common place in malignancy, resulting in the activation of transcriptional regulators like hypoxia inducible factor-1(HIF-1). In order to faciltate neoplasic growth, physiological adaptations appear to be a consequence of HIF-1 expression. Over 50 genes have been discovered to be induced by HIF-1, including: Vascular Endothelial Growth Factor (VEGF) – a promoter of angiogenesis; Glucose Transport 1 (GLUT1) and glycolytic enzymes – critical components in anaerobic respiration; and Erythropoietin (EPO) – responsible for the differentiation of red blood cells.
One of the most inducible HIF-1 genes is carbonic anhydrase (CA). CA has over 14 isoforms which catalyze the hydration of carbon dioxide to carbonic acid, providing a link between metabolism and pH regulation. Decreases in CA9, in a hypoxic microenvironment, are associated with augmented cell death. Transcription of these genes is specifically mediated by the high degree of homology between the Hypoxia Response Elements (HRE), located on the 5’ end of the gene promoters, and the HIF-1 binding sites. Therefore, manipulation of HIF-1 is a potent means of cancer cell growth regulation.
In order for the HIF1 protein to work as a transcription factor, certain conformational changes must occur. During normoxic conditions the amount and activity of HIF1α is limited by post translational modification. Oxygen dependent prolyl- and asparaginyl- hydroxylases are required for the ubiquitination, proteosome targeting and subsequent degradation of HIF1α. Hypoxic conditions restrict prolyl-hydroxylases, resulting in decreased HIF1α turnover and thus increased intracellular amounts. Subsequent phosphorylation of HIF1α allows for its binding to a second subunit, HIF1β. Consequently, the HIF1α:HIF1β dimer combines with CREB (cyclic AMP response element binding protein) to induce activation of the HREs in HIF target genes.
Lipoic Acid Mineral Complex (LAMC) complexes have been extremely effective the more anaplastic the neoplastic cell line. As malignant cells become less differentiated they become more hypoxic with subsequently higher HIF-1 levels. Thus we wished to examine the role of lipoic acid mineral polymer, the irreversibly bound trimer of lipoic acid mineral complex (ALA, palladium, joined to thiamine). Its unique structure allows the molecule to be both water and lipid. The LAMC complex appears to be selectively toxic to the cancer cells, leaving adult neuronal stem cells and HT22 hippocampal cell cultures unharmed. In cancer cells, it has been demonstrated to be an oxidizer of membrane fatty acids, serving as a redox shunt to provide a continuous river of reducing equivalents to protect DNA against oxidation. However, LAMC also acts via complex I of the mitochondria and will oscillate between an oxidized and reduced form as it accepts unpaired electrons and donates them to the electron transport chain. In this experiment, the influence of LAMC on tissue hypoxia and subsequent HIF-1 levels were examined to provide further explanation as to the means of LAMC-induced therapy.
Summary of outcome studies by Dr. James Forsythe, Century Wellness Center
Total Survivors With Integrative Therapy at 64 Months 690/950 = 72%
Standard 3 week protocol 5 day a week Integrative Protocol.
- First Outcome Study: Poly-MVA only with IPT Therapy – 225 Patients 6-year Overall Survival (OS) rate of 32%
- Second Outcome Study: Poly-MVA / IPT / FIP Therapies – 500 Patients 5-year Overall Survival rate of 39%
- Does IV Loading Dose of Poly-MVA Make A Difference?
- Overall Response Rate on oral Poly-MVA only at 30 month mark = 30/75 (40%)
- Overall Response Rate on IV + Oral Poly-MVA at 30 month mark = 45/75 (60%)
- Loading dose difference = 20% improvement at 30 months
Summary: Outcome-based investigations
- Clinical Oncology “Outcome Based” investigations on metastatic cancers of multiple origins.
- The investigation was voluntary and not double-blinded or placebo controlled.
- The major parameters included: CR – All Clinical disease in Remission,
- PR – Greater than 50% reduction in tumor mass/markers,
- SD – Less than 50% reduction in tumor mass/markers.
- 56% at 30 Month and 38% at 60 Month overall response rate (ORR) with CR +PR +SD.
- The ORR in patients on Poly-MVA only was 40%.
- The ORR in patients on chemotherapy + Poly-MVA was 60%.
- These results showed significant improvement over historical controls and far less side effects.
Conclusions of investigation
Poly-MVA is a safe (both orally/IV) and extremely effective supplement for support and palliative assistance in stage IV cancer patients, either with or without concomitant chemotherapy.
The safety profile is excellent and there were no treatment related deaths or any significant adverse reactions or negative interactions with chemotherapy or hormonal treatments.
The best responding tumors were: 1) Prostate, 2) Breast, 3) Lung, 4) Head/Neck, 5) Colorectal, and 6) Hematological. Results show an improved Overall Response Rate over historical controls.
An IV loading dose of Poly-MVA confers a 20% improved ORR in this investigation.
Homeopathic oncology philosophy
In Stage IV cancers of any origin, improvement in Quality of Life issues is directly proportional to improvement to overall response rate. Even stable disease can be tolerated and metamorphosed into a chronic livable condition.
This is true provided that this improvement is not gained at the expense of toxic chemotherapy or radiation therapy leaving the patient with many of the following adverse side effects:
- Chemo Brain Syndrome
- Dermatoses
- Painful Neuropathies
- Cardiomyopathies
- Renal Failure
- Hepatic Failure
- Severe Pancytopenias
- Pulmonary Fibrosis
- Devastating Fatigue, Anorexia
- Wasting Syndromes
- Death
These studies and the various case reports continue to confirm that the “cure/kill” approach to cancer treatment is not the only solution and more research is needed.
Common questions
I am frequently contacted at my laboratory, on the radio, or approached at conferences with common questions regarding Poly MVA.
A number of people are under the impression that Poly MVA is merely a cocktail of alpha-lipoic acid, thiamine, minerals, riboflavin, cyanocobalamin, formyl-methionine and acetyl-cysteine. There is no free lipoic acid or mineral in Poly MVA, rather it is bound irreversibly with thiamine. Therefore, comparisons to free palladium or lipoic acid are not equal. This was done by Dr. Garnett to create a metallo-polymer that is both fat and water soluble. Furthermore, it is prepared in a unique fashion so it does not metabolize, dissociate, and liberate any of the components.
Poly-MVA/LAMC complex have undergone extensive study (Calvert Laboratories, Inc; Pharmakon USA, Inc.). The toxicology was conducted both intravenously and orally with LAMC. Mice were administered doses of 5,000 mg/kg (a typical human dose is 20 mg/kg). No deaths or signs of organ damage occurred in the test animals. It was concluded that the LD50 of LAMC exceeds 5,000 mg/kg. The Ames test was conducted by the same independent lab and demonstrated not to cause any mutations. LAMC was also studied for its’ effectiveness in halting the growth of glioblastoma cells in vivo. Tumors were allowed to establish and mice were divided into 8 groups of 10. Four groups were given daily intravenous (IV) doses LAMC or placebo; four groups were given intraperitoneal doses of .05, 1.0 or 2.0 mg per mouse for a total of four weeks and tumor volume was measured throughout the study. Compared to the controls who received no LAMC, mice receiving the test material orally or intravenously at 0.5, 1.0 or 2.0 mg had a significantly reduced growth of the glioblastoma (50% or greater reduction in tumor size).
Palladium is a precious mineral and quite expensive. Why was it used? Dr. Garnett discovered during his electrochemistry studies that DNA (and other biological cellular entities) has select electrical frequencies that it resonates at. After testing thousands of chemicals, Dr. Garnett found that only a limited few metals shared the same resonance frequencies with DNA. This characteristic facilitates electron flow between them. This is analogous to the propulsive energy provided to a surfer by a wave. If the surfer is in “sync” with the wave he can ride it all the way in to the beach. However, if he or she isn’t, they will crown right over the top of the wave. Palladium is important since this is the only one that Dr. Garnett could “connect” with the lipoic acid and thiamine to form this irreversible crystal polymer.
Why are the other components added to the dietary supplement? Most people feel the other components are added without regard to the LAMC complex, but this is not true. Any molecule that transfers electrons has the ability to generate heat. The other cofactors that complete the PolyMVA play the role in buffering actions of LAMC.
Is Poly MVA just a super free radical scavenger? This was my initial thought after my first transient global ischemia experiments with the LAMC complex in Poly MVA. However, the LAMC complex is a liquid crystal polymer. There is extensive data supporting the dramatic redox potential of polymers versus monomolecular structures, such as vitamins. Any redox molecule can certainly absorb electrons, but it also donates them. Dr. Garnett’s electrochemistry papers demonstrate LAMC’s significant redox potential versus its monomolecular competition. After my initial ischemia research findings, I sent some Poly MVA to Brunswick Labs, Inc. (Wareham, MA) for an ORAC analysis. An ORAC assay measures the oxygen radical absorbance capacity of a compound as compared to Trolox (vitamin E). The table below demonstrates the potent anti-oxidant capacity of Poly MVA (expressed as Trolox equivalent per gram):
- Vitamin A = 1.6 (2,800)
- Vitamin C = 1.12 (1,890)
- Vitamin E = 1.0 (1,700)
- Melatonin = 2.04 (3,468)
- Lipoic Acid = 1.4 (2,400)
- Poly MVA = 5.65 (9,605)
Why is this supplement often credited or associated with providing energy? While Poly MVA does indeed have the ability to be a highly effective free radical scavenger,its ability to donate electrons to the mitochondria of the cell is critical to explaining its dramatic benefits. Documented clinical outcome based evidence of the reports of additional energy, led to my early hypotheses regarding its possible benefits in stroke and ischemia. Following an interruption of blood flow to any tissue, in my particular case it is the brain, there is deprivation of oxygen and glucose. Providing an alternative energy source can maintain the integrity of the electron transport chain within the mitochondria. The LAMC complex was demonstrated, by Dr. Garnett, to shuttle electrons to oxidized DNA, however, this energy flow does not appear to proceed directly to DNA. By conducting a competition assay with lipoic acid, which works at complex I of the mitochondria as a cofactor as pyruvate is converted to acetyl CoA, one can attenuate the beneficial effects. This is critical since mitochondrial health is a major concern during myocardial and cerebral ischemia. By providing this alternative energy source, the electron transport chain components do not readily dissociate (coenzyme Q-10 = ubiquinone; cytochrome C). In a normal cell this would obviously provide a boost, but serve as a supplement to an ischemic cell.
Can Poly MVA be taken with other vitamins and free radical scavengers? Since LAMC is a highly efficient redox molecule, normal daily recommended values of vitamins have not been of consequence in our laboratory studies. However, excessive high doses of anti-oxidants may attenuate Poly MVA’s benefits and need to be done according to the doctors protocols. As mentioned above, administration of alpha lipoic acid in our competition assay hindered the redox benefits of Poly MVA. However, alpha lipoic acid alone only offers a fraction of the ischemic protection offered by the polymer.